Research
In the Marsden lab we aim to better understand the processes underlying neurological disease by uncovering genetic and environmental factors that impact the development of neural circuits and behavior.
Using zebrafish as our model system, we take a variety of experimental approaches including gene expression analysis, high-throughput behavioral testing, 3- and 4-D analysis of neural circuits, simultaneous imaging of neuronal activity and behavior, and optogenetic techniques.
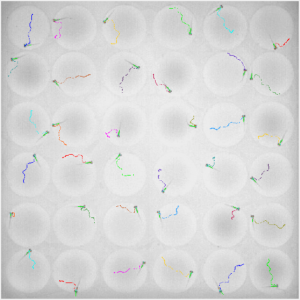
Genetic regulation of behavior thresholds
We are exposed to a constant stream of sensory stimuli, yet only some of them are relevant to us. So how do we decide which ones to respond to? For example, how loud must a sound be to cause you to startle? We have to set a behavioral threshold to filter out innocuous or irrelevant stimuli and focus on those that are important. This filtering process is often disrupted in individuals with autism or anxiety disorders, as many are hypersensitive to auditory stimuli and are easily startled by low-intensity sounds. Such sensory over-responsiveness can also follow visual or tactile stimuli, as many people that suffer from migraines experience hypersensitivity to light, and those with allodynia experience normally innocuous tactile or thermal stimuli as painful. The goal of this work is to identify genetic regulators of these behavioral thresholds and find out how they influence the formation and/or function of the underlying neural circuitry.
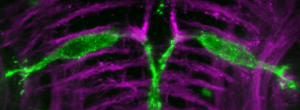
When they are just a few days old, zebrafish larvae perform stereotyped responses to sound, light, and tactile stimuli. And because they are just a few millimeters long, we can simultaneously test the behavior of many zebrafish larvae in response to these stimuli using high-speed cameras to record their movements. This high-throughput approach allows us to perform chemical and genetic screens to discover molecular pathways that regulate behavior in an unbiased manner.
To identify genes that control the threshold for the acoustic startle response, we induced random mutations throughout the zebrafish genome. We then isolated mutant lines that startle to low-intensity acoustic stimuli. One of these mutants is called triggerhappy.
triggerhappy mutants have a premature stop mutation in the gene cyfip2 (cytoplasmic Fragile-X Metal Retardation Protein interacting protein 2), which regulates the actin cytoskeleton. We are actively working to understand how loss of cyfip2 alters brain function to control the startle threshold using approaches like the simultaneous imaging of neuronal activity and behavior shown in this video. In this movie, one of the startle command-like neurons, the Mauthner cells, has been genetically labeled with an activity reporter, GCaMP6s. Using a spinning disc confocal microscope we can record the activity of this neuron in response to sound (left) while simultaneously recording the behavioral response of the fish’s tail with a high-speed camera (right). Low-intensity sounds don’t cause the fish to startle, but they do produce subthreshold, synaptic responses in the Mauthner cell’s lateral dendrite. A high intensity sound triggers a massive increase in Mauthner cell brightness, indicating it fired an action potential and caused the fish to startle. This technique allows us to identify altered patterns of activity caused by the loss of genes such as cyfip2.
We can also use this method to study neuronal activity in response to tactile stimuli. Poking the fish on the tail causes only the Mauthner cell on the side that was touched to fire, and elicits a similar startle response to the side opposite the stimulus shown in this video.
Understanding the behavioral symptoms of CHARGE Syndrome
CHARGE syndrome is a rare, multi-system disorder that affects approximately 1 in 10,000 newborns. The most common symptoms include ocular coloboma, choanal atresia, heart defects, genital abnormalities, ear malformations, and an array of neuro-behavioral difficulties. Sensory under- or over-load, motor impairments, enhanced pain, sleep disorders, attention deficit hyperactivity disorder (ADHD), obsessive compulsive disorder (OCD), intellectual disability, anxiety, and autism are all frequently observed in CHARGE. Treatments for these behavioral symptoms are limited, however, highlighting the need to better understand the mechanisms underlying these defects in order to develop new therapeutic approaches. Two-thirds of CHARGE cases are caused by loss-of-function mutations in chd7, which encodes a DNA-binding, ATP-dependent chromatin remodeling protein. We have used CRISPR/Cas9 to generate a zebrafish model of CHARGE syndrome, and we are currently defining the morphological and behavioral impacts of chd7 mutations. We are also using brain-wide imaging and combined proteomic and transcriptomic approaches to identify cellular and molecular pathways that drive behavioral dysfunction in this CHARGE model.
Defining links between cyanotoxin exposure and neurodegeneration
Exposure to cyanotoxins produced in harmful algal blooms is linked with neurodegenerative diseases such as Amyotrophic Lateral Sclerosis (ALS), but little is known about the combined effects of exposure to mixtures of cyanotoxins. We have found that a binary mixture of two highly prevalent cyanotoxins, BMAA and Microcystin LR, synergistically alters behavior of larval zebrafish (Martin et al 2021). Using unbiased shotgun proteomics we have also found that this mixture dramatically increases the abundance of TDP-43, a protein whose cytoplasmic aggregates are the pathological hallmark of ALS. We are currently working to determine the long-term effects of developmental exposure to this and other mixtures of cyanotoxins using a combination of behavioral, imaging, genetic, and pharmacological approaches.